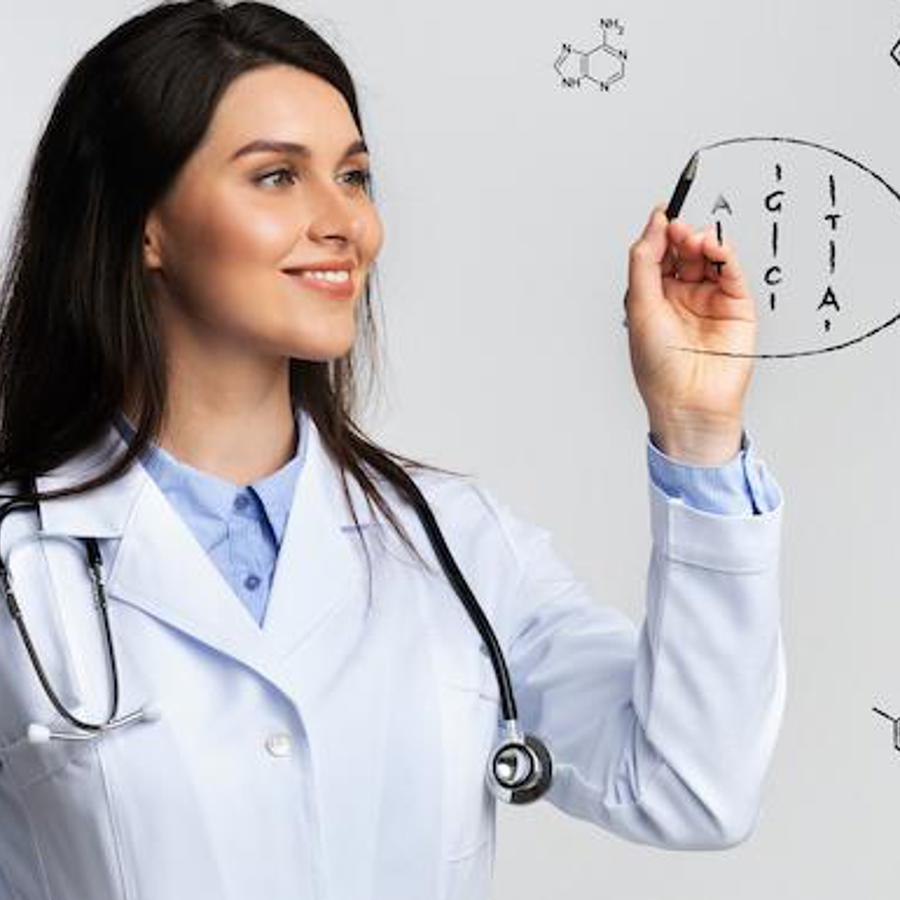
How could you make a lab designed gene?
June 19, 2008
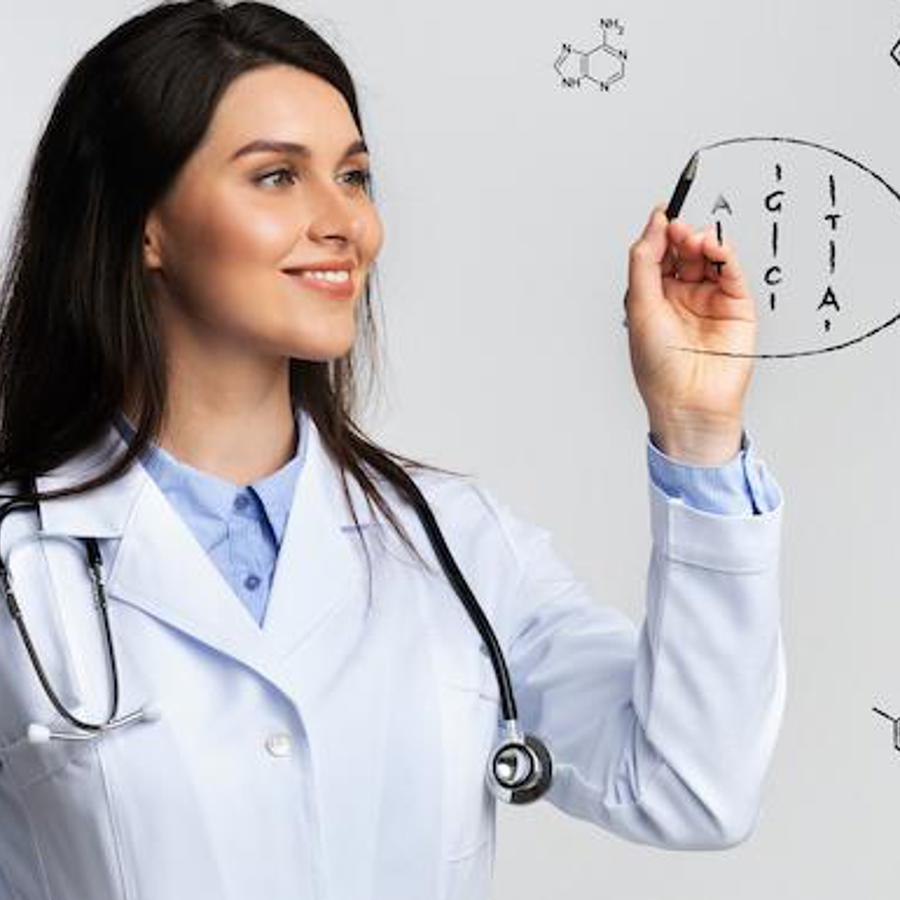
- Related Topics:
- Synthetic biology,
- Biotechnology,
- Genetic engineering
A middle school student from California asks:
"How could you make a lab designed gene? Is it possible?"
Yes, it's definitely possible to make a lab-designed gene. In fact, scientists have been doing it for years! The first lab-designed gene was made thirty-five years ago in 1972.1
Scientists first started making synthetic genes by cutting and pasting parts of different genes together. This "simple" method hasn't really changed over the years. It is still the most common way of making a lab-designed gene.
But technology has improved over time. So scientists are also able to create genes entirely from scratch.
You might be asking yourself why scientists waste their time making designer genes. Turns out it's not a waste of time at all!
Lab designed genes are not only used by scientists to study biology. They are also used to make life-saving drugs like insulin. More recently they've also been used to create genes used in gene therapy and genetically modified foods.
Let's learn more about how lab-designed genes are made and why. But before we can talk about making a gene, we have to understand what a gene is and what it does.
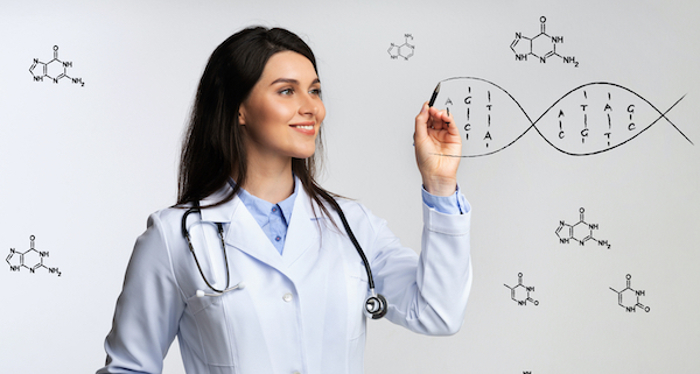
Making Genes in a Lab
A gene is a piece of DNA that has instructions for making a protein. These instructions also include when and how much protein to make. Proteins are molecules that are involved in almost everything a cell does.
The reason a scientist designs a new gene is usually because they want to change something about the old gene. They want to change what a protein does, where it is found or how much of it gets made. They might also change whether a protein gets made in a person, a bacterium, a mouse, or whatever.
When a scientist changes a gene it is called recombinant technology. All this really means is combining DNA in new ways.
As I said earlier, there are two main ways for scientists to make recombinant DNA. The most common is by cutting and pasting together genes or parts of genes. The second is creating a synthetic gene directly from DNA.
For example, think of a gene as words where the letters are DNA. To make the word "basketball" you can cut and paste the words "basket" and"balloon" together. Or you could use the letters b, a, s, k, e, t, and l to make the word from scratch.
In both of these examples we're recombining letters to make a new word. One method uses pre-existing words to make a new word. The other makes a word from individual letters.
This is the same as recombining DNA to make a new gene. So how exactly can you recombine DNA to create a gene? Let's quickly review the structure of DNA to understand how this is done.
DNA is a double stranded molecule. The two strands are each made up of a chain of nucleotides, abbreviated A, G, T, and C.
The order of these letters has the instructions for how, when, where, and how much of a protein to make. One way to make a synthetic gene is to recombine these instructions.
Mixing and Matching
For example, let's say we wanted to make insulin in bacteria. To do this, we need to combine the human instructions for how to make the protein with bacterial instructions for where and how much to make.
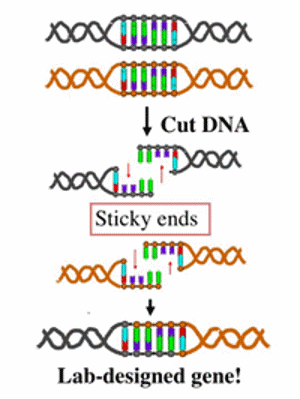
Scientists can do this because of a special property of DNA — each nucleotide only pairs with one other nucleotide. T pairs with A and G pairs with C. For example, if you have one strand that is A-T-C-G, the opposite strand will be T-A-G-C.
Scientists can take advantage of this by using proteins found in bacteria called "restriction enzymes." These enzymes are like scissors that chop up double stranded DNA. But different enzymes cut the DNA at different specific sequences.
For example, one enzyme called EcoRI cuts only GAATTC. Another, BamHI, cuts only GGATCC. So if you know the DNA letters of your gene, then you can use the right enzyme to cut it in the right place.
Most restriction enzymes also cut DNA in such a way that it creates a sticky end. What this means is that it is easy to stick an EcoRI cut end to another EcoRI cut end, but hard to stick it to a BamHI end, because A pairs with T and G pairs with C.
So if you cut two different pieces of DNA with the same restriction enzyme, you would get the same sticky end. Scientists use these sticky ends to glue together two different DNA molecules. This ends up making a recombinant gene.
Making a Gene From Scratch
The other way we talked about making a recombinant gene is to do it from scratch. What scientists do is make one strand at a time and then put the two back together.
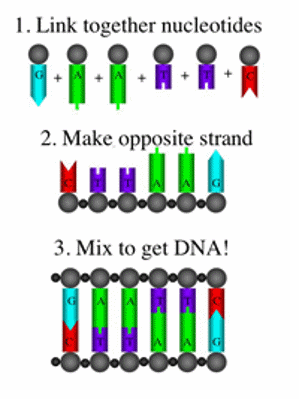
They use chemical reactions to link together the nucleotides A, T, G, and C in whatever order they want. They then make the opposite strand so that A pairs with T and G pairs with C. By mixing the DNA strands together they can create a synthetic gene.
Using this method it is technically possible to make a DNA molecule up to 7,000 nucleotides long. That is large enough to make an entire gene.
But it is both time consuming and very expensive to make DNA this way. Which is why most scientists use the cutting and pasting method to make lab-designed genes.
Using Lab Made Genes
Now you've learned all about genes and how scientists can make them in a lab. Let's focus now on the why. What are lab-designed genes used for?
One reason is for studying science. For example, many biologists study what certain proteins do in our cells. And they've found a way to SEE the protein inside the cell to get an idea of how it's working!
To do this, scientists use glowing proteins from jellyfish. One is called GFP- green fluorescent protein. Scientists have used recombinant DNA technology to create many new versions of this gene. Now they have proteins that are all the colors of the rainbow.
So how can scientists use these glowing proteins? They cut and paste the GFP gene onto the gene of the protein they are interested in studying. By putting this new gene into cells, scientists are able to look at their glowing protein.
Another use for lab-designed genes is making life-saving drugs. One example is insulin.
Insulin is a protein made by our bodies to help us control the sugar in our blood. People with a certain type of diabetes cannot make their own insulin. So they need to inject themselves every day with insulin to stay healthy.
Scientists took a copy of the insulin gene from humans and put it into bacteria. The bacteria now make lots of insulin protein from the DNA. So the bacteria end up being an insulin-making factory!
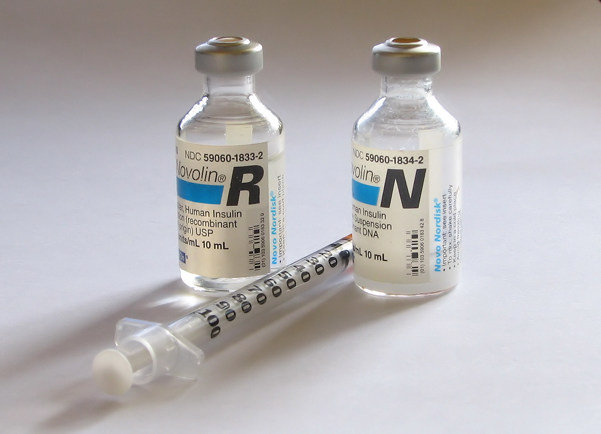
And insulin isn't the only drug being made this way. Other examples include medicines like EPO or growth hormone. Scientists are also using recombinant DNA to make drugs in bacteria that can fight malaria or try to cure peanut allergies. And there are many more examples.
In the future, the possibilities for recombinant DNA are endless. Just this past year scientists were able to make the entire DNA genome from a specific type of bacteria completely from scratch. Even though this bacteria has a pretty small genome, that's still 582,970 nucleotides long!
Knowing how to recreate the DNA from bacteria means scientists can now make designer organisms, not just designer genes. And this is just the beginning.
Recombinant DNA will continue to improve our crops, make better medicines, and do things we haven't even thought of yet.
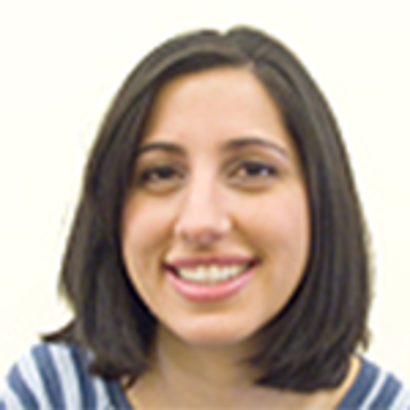
Author: Jackie Benjamin
When this answer was published in 2008, Jackie was a Ph.D. candidate in the Stanford Department of Cancer Biology, studying cell-cell adhesion and membrane dynamics in James Nelson’s laboratory. Jackie wrote this answer while participating in the Stanford at The Tech program.